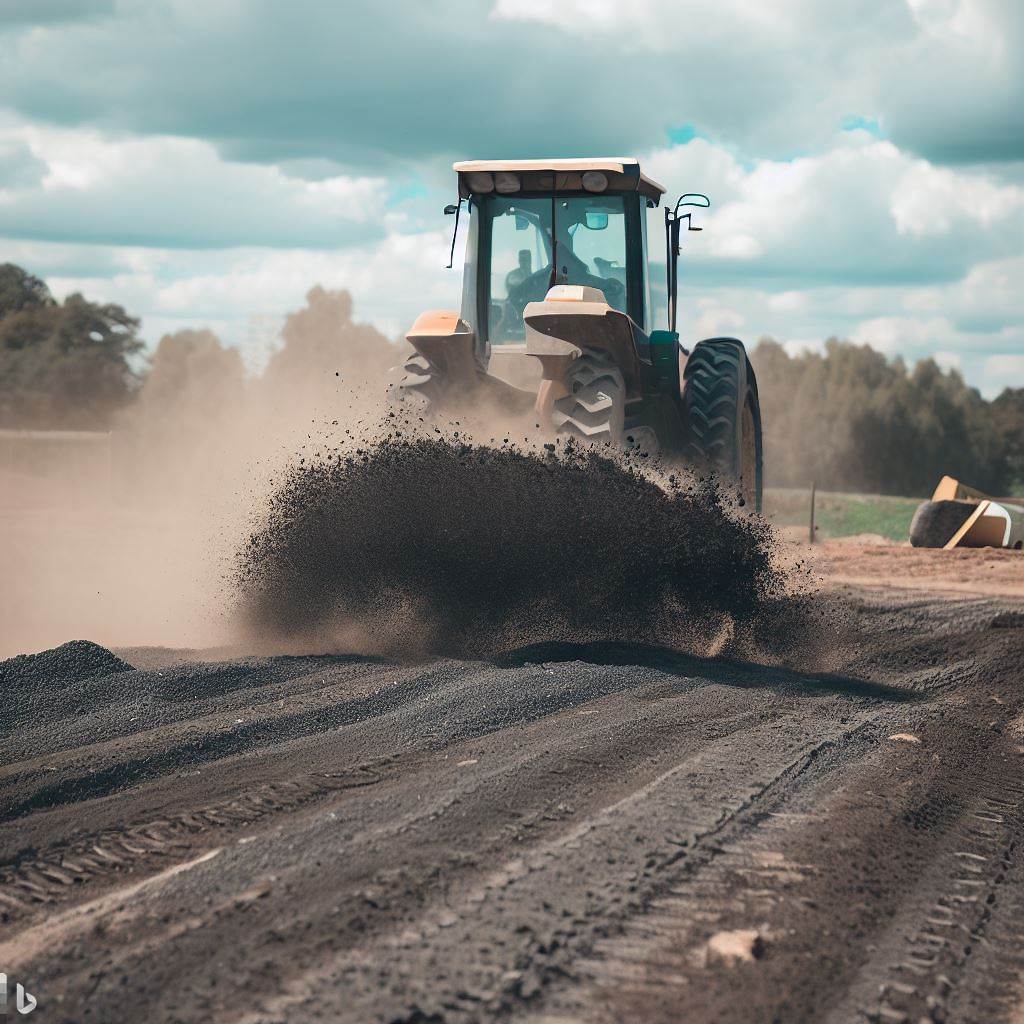
Startups Turn to Global South for Carbon Removal as They Discover the Money Behind Rock Weathering
The article highlights the role of negative emission technologies (NETs) in conjunction with decarbonization efforts and explores the potential of Enhanced Rock Weathering (ERW) as an effective carbon removal solution. Additionally, it discusses the environmental benefits of ERW and its competitiveness compared to other NETs. The article showcases some climate startups that have received lucrative funding and concludes by prospective value sharing of carbon credits with basalt miners and farmers in India.
Demand for carbon credits by 2050
In total, 196 countries agreed to keep average temperature rises to 2°C, ideally 1.5°C, above pre-industrial levels, making the Paris Agreement a legally binding international treaty on climate change in 2015. According to an ongoing temperature analysis led by scientists at NASA’s Goddard Institute for Space Studies (GISS), the average global temperature on Earth has already increased by 1.1°C since 1880. About 75% of this temperature increase was noticed after 1975. To limit global warming to 1.5°C, greenhouse gas emissions must peak before 2025 at the latest and decline by 43% by 2030, as per the United Nations Framework Convention on Climate Change (UNFCCC).
According to model forecasts, rapid decarbonization may be insufficient to limit global warming without the simultaneous deployment of negative emission technologies (NETs). In other words, even if every country cut its CO2 emissions to zero, our globe would not cool off. To attain global temperature at the level of the preindustrial era, we will also need to remove a certain amount of CO2 from the atmosphere that has been emitted in the past and will be emitted in the future, as we cannot practically achieve zero emissions in various business operations due to technological limits.
By 2050, the Intergovernmental Panel on Climate Change (IPCC) suggests removing 10 billion metric tons (10 Giga tons) of CO2 from the atmosphere per year. This amount of CO2 taken from the atmosphere must be locked up geologically so that there is no possibility of leakage or re-emission into the atmosphere.
While the Science Based Targets Initiative (SBTi) and several other initiatives were already in place to drive governments and corporations toward CO2 emission reduction and decarbonization of business processes, the need to remove CO2 from the atmosphere has also fuelled the search for negative emission technologies (NETs). Recently, firms that have committed to net-zero targets as per SBTi and other pledges such as Amazon’s ‘Climate Pledge’ have been funding a growing number of NET startups. The Climate Pledge compels businesses to achieve net-zero carbon emissions by 2040, ten years ahead of the Paris Agreement, by installing decarbonization technology and also offsetting any residual emissions. For example, if an automobile sector company needs to reduce its carbon emissions by 90% by 2045 to achieve net-zero targets under the SBTi approach, the Climate Pledge requires the company to prepone the target for 90% reduction to 2040 and also offset the remaining 10% of emissions after 2040 that cannot be eliminated due to technical constraints.
As a result of the present scenario and forecast for 2030 and 2050, the requirement to offset remaining emissions is expected to increase demand for carbon credits. In a 2021 report titled “A blueprint for scaling voluntary carbon markets to meet the climate challenge”, the Taskforce on Scaling Voluntary Carbon Markets (TSVCM), sponsored by the Institute of International Finance (IIF) with knowledge support from McKinsey, estimates that demand for carbon credits could increase by a factor of 15 or more by 2030 and by a factor of up to 100 by 2050. Overall, the carbon credit market might be valued at more than $50 billion by 2030 (McKinsey & Company).
Negative Emission Technologies and ERW in Focus
As a result of the present scenario and forecast for demand for carbon credits, a large number of pilot-scale projects have begun around the world to develop and commercialize “Negative Emission Technologies (NETs),” such as Enhanced Rock Weathering, Biochar, Carbonated Building Materials, Woody Biomass Burial, and Geological Storage of Direct Air Carbon Capture.
A quick rundown of these technologies
1) Biochar is created by pyrolyzing biomass or biowaste. Pyrolysis is the process of heating an organic material, such as biomass, without the presence of oxygen. Because there is no oxygen present, combustion does not take place; instead, the biomass thermally decomposes into combustible gases and biochar. The majority of these combustible gases can be condensed into pyrolysis oil (bio-oil). Biochar is a very stable, solid form of carbon that can survive in the soil for thousands of years, making it an attractive solution for scalable carbon removal. The biochar can also be utilised in various industrial processes as an absorbing agent before locking it geologically to store the carbon.
2) Carbonated building materials are CO2-negative concretes that absorb more CO2 than they emit in their production process. CO2 is chemically bonded and mineralized into the building ingredient during the hardening step.
3) Woody mass burial, as the name suggests, is the concept of burying woody biomass under conditions that hinder biomass breakdown and can maintain those conditions for at least 100 years.
4) Geological carbon storage entails technology such as the direct injection of CO2 into deep geological formations and oil and gas reserves to improve oil recovery. As a result, new technologies and startups are emerging to filter carbon from atmospheric gases and lock it into geological formations. It should not be confused with Carbon Capture and Utilization (CCU) technologies, which generally refer to the capture of carbon from industrial processes and carbon-rich flue gases before releasing them into the atmosphere, and then reusing this carbon in industrial processes to replace the need for fossil fuels as a carbon source. While CCU is required, it is categorized as a “decarbonization” technology rather than a “negative emission technology,” which entails removing carbon from the atmosphere and storing it in geological formations and other forms for the long term.
5) Enhanced rock weathering (ERW) is simply the acceleration of the natural process of rock weathering, which takes hundreds of millions of years. In rock weathering, carbon dioxide reacts with rainwater to generate carbonic acid. When this dilute acid falls on Earth, it reacts with the rocks and soil, forming carbonate, which is safely stored for thousands of years.
ERW technology, a boon for carbon removal
The enhanced rock weathering method is straightforward, as it entails finely grinding the selected silicate minerals and applying them to land surfaces such as agricultural fields or forest areas. This can be accomplished by physically or mechanically spreading the substance or by using techniques such as aerosol spraying. When minerals are applied to the land, they react with CO2 and water in the plant’s root zone, forming carbonates and bicarbonates. Carbonate minerals are soil constituents that stably store CO2. The bicarbonates are dissolved in soil water and can eventually leach and reach the bottom of rivers, streams, and oceans, where they get stored for a long time. As a result, we shorten the timeline of rock weathering’s environmental advantages from millions of years to a few decades. Carbon storage through ERW is assured for thousands of years in both forms, with no possibility of reversibility owing to natural or human activities.

Rate of carbon dioxide removal by ERW:
The Carbon dioxide Removal Rate (CDR) for a given geographical area is the amount of CO2 that ERW can remove from the atmosphere every year. This rate is determined mainly by three factors: (a) the specific carbon sequestration potential of the rock; (b) the amount of rock scattered across the land area; and (c ) the proportion of rock that weathers each year. The maximum quantity of CO2 absorbed from the atmosphere by silicate dissolution is determined by the cation flux (mostly Ca2+, Mg2+, K+, and Na+), which is charge balanced by the creation of HCO3 (Hotchkiss et al. 2015).
Mafic rocks, such as basalt, can remove up to 0.3 t CO2 from the atmosphere per t basalt (Strefler et al. 2018). How much basalt powder is dissolved in the soil every year also depends on the grain size of the powder. According to research, the grain size of ground rock should be less than 100 microns (m). Improved weathering is found when grain sizes in basalt dust was reduced to 10 microns. Within 10 years, the amount of basalt powder dissolved was around 16% (100 microns), 55% (10 microns), and 99.9% (1 micron). This equates to a net CO2 removal of 0.045 t CO2 per t of 100 micron-size basalt powder and 0.153 t CO2 per tonne of 10 micron-size basalt powder (Rinder et al. 2021).
It is mentionable that rock grinding to finer levels contributes to the overall energy requirement and carbon dioxide emissions of ERW, and transporting and spreading grain size less than 50 m can also contribute to practical and health difficulties due to air-borne dust. So, research on the optimal benefits of ERW in location-specific conditions in India is required. There are several studies conducted in various conditions to assess the CDR by applying volcanic rocks. One experiment that added basalt powder to slightly acidic clay-loam soil found that it removed a total of 4 t CO2 per ha over 5 years (Kelland et al., 2020). In another trial, the CDR potentials were between 1.3 and 8.5 metric tons of CO2 per ha after 15 years of weathering for a single baseline application rate of 50 t per ha of crushed basalt (Lewis et al. 2021).
Global carbon dioxide emissions from fossil fuels and industry were 37.12 billion metric tons (GtCO₂) in 2021. The potential for permanent carbon drawdown and agricultural co-benefits of ERW make it an appealing mitigation strategy, especially in equatorial and near-equatorial geographies like the Global South, where soil pH, temperature, and moisture conditions are ideal for the technology (Boudinot et al. 2023). By using ERW, China, India, the United States, and Brazil alone have the potential for 0.5 to 2 gigatonnes of carbon dioxide (CO2) removal from the atmosphere per year, with extraction costs ranging from US$80 to US$180 per tonne of CO2. If continued over 50 years, the total amount of CO2 removed globally might range between 25 and 100 Gt CO2 (Beerling et al. 2020).
Global carbon cycle modelling based on ensemble Representative Concentration Pathway (RCP) projections of twenty-first-century climate change (RCP4.5, medium-level mitigation pathway) indicates that enhanced weathering could reduce atmospheric CO2 by 300 ppm by 2100, primarily depending on a silicate rock application rate of 5 kg per m2 per year (Taylor et al. 2016).
Environmental Benefits of ERW:
Aside from carbon reduction, enhanced rock weathering provides several unique environmental benefits. One of the most notable benefits of ERW is its long-term carbon storage capacity. Unlike other carbon removals methods such as carbon capture and storage (CCS) or biomass use, the captured carbon in ERW is permanently stored in soil or underwater with no major chance of re-entering the atmosphere.
This process produces calcium and magnesium ions, which aid in soil remineralization. Soil remineralization can improve soil structure, nutrient content, and water retention capacity, resulting in higher agricultural yields and less reliance on chemical fertilizers. This method also helps to decrease soil erosion and improve water quality by lowering fertilizer leaching. Rock weathering supplies nutrients to plants and fungi, and it is well-known that microbes and fungi can accelerate mineral dissolution rates. (Hartmann et al. 2014).
ERW does not require any complicated technicalities and is simple to apply; all that is required is to sprinkle the mineral dust across the farm ground and plough it before irrigation.
Another advantage is that the most suitable land types for ERW are acidic arable and forest lands, as well as degraded plantation sites. Warm and humid environments are best suited for ERW. Particularly in India, Brazil, Southeast Asia, and China, where over 75% of the worldwide potential of ERW can be achieved (Strefler et al. 2018).
Competitiveness of ERW with other NETs:
The carbon footprint of the captured CO2 is shown to be mainly dependent on the carbon footprint of the electricity supply. ERW is found to be competitive with other large-scale CDR strategies in terms of energy, land, and water requirements and has additional advantages over some of them by requiring less energy (e.g., in comparison to direct air capture) and less water (e.g., in comparison to afforestation), while supporting agricultural production rather than competing with it (Eufrasio et al.).
Appropriate rock in India for carbon removal
Various types of silicate rocks that have high levels of calcium and magnesium are ideal for ERW. Basalt is the most common rock on the planet and has these properties. Basalt is more commonly available and less expensive in India than other rocks containing significant levels of silica. Basalt columns are known to occur as a result of the rapid cooling of volcanic lava on the earth’s surface in the presence of water. In west-central India, the Deccan Traps comprise a huge igneous province. It is one of the world’s largest volcanic features. It is made up of multiple layers of solidified flood basalt that are on average 2,000 meters thick and cover an area of around 500,000 square kilometers.

In India, there are numerous basalt mines. Basalt dust may currently be purchased in bulk in India for INR 6,000 to 7,000 per tonne. Misrauli, near Kota in the state of Rajasthan in India, is one region where many black basalt quarries are under operation. There are some grinding mills installed for other purposes in and near Kota City that can grind basalt up to 100 micron-fine powder for trials. Given the high demand for basalt dust, its price is anticipated to rise worldwide shortly (Abramoff et al. 2021). For the ERW application, we do not need a good-quality basalt column to crush dust. We only need dust or gravel removed to waste during the basalt mining. This gravel-sized basalt at the mine site hardly has any price. Presently, by optimising the purchase of raw material and grinding, one can optimise the cost of landed basalt dust within the price range that can fit into the global startup’s mission of CO2 removal at less than $100 per ton by 2035. Support from India can help global startups to improve their vision from 2035 to 2030. Later, when we start large-scale grinding for large-scale ERW projects in India, the economics of scale will come into play to optimise the cost of basalt dust landed at fields.


Startups Seeking Funding in ERW Space
While governments and corporations around the world strive for net zero emissions by incorporating decarbonization technologies into business processes, a growing number of businesses are emerging to provide solutions in the space of atmospheric carbon dioxide removal.
- Everest Carbon is committed to using ERW to help combat climate change. Removing plans to remove 1 billion tons of CO2 by 2035 for less than $100/t. The company leverages its unique selling proposition (USP) to not only remove CO2 from the environment but also to improve soil health and encourage sustainable agriculture. They hope to make increased rock weathering an economically viable and scalable option by using developments in grinding technology, deployment methodologies, and logistics.
- Vesta.earth, is a pilot initiative for a gigatonne-scale CO2 removal project company. Vesta’s purpose is to aid in the reversal of climate change. Vesta is devoted to conducting a thorough study into the underlying dangers and impacts of Coastal Carbon Capture (CCC).
- InPlanet is a German-Brazilian firm that uses ERW to combat climate catastrophe. Niklas Kluger and Felix Harteneck created InPlanet in 2022 after discovering the potential of Enhanced Rock Weathering (ERW) for carbon removal in the tropics during a trip to Brazil. InPlanet expands research collaborations with the Universities of Sao Paulo (ESALQ), Brasilia (UNB), and Newcastle. This GreenTech firm recognizes that offsetting carbon from unavoidable emissions is a critical step toward protecting life on Earth. It just received €1.2 million in new funding to develop its solution in the tropics, intending to create a carbon-zero future.
- Lithos Carbon aims to capture 10,000 tons of carbon within 2023. This US-based startup makes farmland into carbon removal centres using volcanic rocks. Besides, carbon removal, this startup is measuring the crop yield benefits and bringing as much revenue as possible to farmers from these carbon credits sold.
- UNDO, a London-based climate tech startup spreading volcanic rock dust on farms to capture carbon in the soil, has secured $12m in funding from Lowercarbon Capital, AENU, and carbon removal purchaser Stripe.
Institutions working on NETs in India:
Due to the number of startups entering this field and making enhanced rock weathering a commercial in the space of NETs, it is likely to have a significant impact on global carbon emissions. Governments, research institutions, and private entities are collaborating to optimize the technology, address the challenges, and establish robust policies that support its implementation.
In India, the government has set up centres of excellence (CoE) in two educational institutions. One is the National Centre of Excellence in Carbon Capture and Utilization (NCoE-CCU) at the Indian Institute of Technology (IIT) Bombay, Mumbai. Another CoE is the National Centre in Carbon Capture and Utilization (NCCCU) at the Jawaharlal Nehru Centre for Advanced Scientific Research (JNCASR), Bengaluru. These CoEs are being set up with support from the Department of Science and Technology, Government of India. These CoEs are mandated to facilitate the capture and mapping of current R&D and innovation activities in the domain and also develop networks of researchers, industries, and stakeholders with coordination and synergy between partnering groups.
Scalability and Policy Considerations of ERW
Startups registered in other countries and taking the lead in ERW are set to apply the process in India and earn carbon credits. Framers and landowners may lose a genuine share in value creation in the absence of awareness and clear guidelines for all stakeholders.
While there are several studies that show initial improvements in crop yield, there is very little data on the annual application of rock power and the long-term impacts of ERW on crop yields. A few studies also found reduced yield in response to high application rates of some minerals used for ERW, indicating potential long-term reductions in yield with the continued application (Haque et al., 2020). Hence, there is also a risk of land contamination if ERW is applied without proper scientific studies specific to the land types in India, which may change from field to field in certain cases.
What is important to address as soon as possible is setting Indian standards for the application of basalt dust with specifications for its composition in agricultural fields and forest land. This will avoid the risk of soil toxicity due to the application of untested rock dust containing toxic elements in high concentrations. Publication of such standards will also give companies the confidence to do business in the ERW space.
Indian ventures in this field may also lose profit margins by paying monitoring, reporting, and verification (MRV) and registry fees to agencies, which are nothing but private companies incorporated in foreign countries and operating in the voluntary carbon market. Besides regulatory controls on technical aspects of basalt application in fields, the CoEs established by the government in India is also expected to initiate and control aspects of the MRV process for carbon removal and the registry of carbon credits from ERW in India.
References
Abramoff, R.Z., Georgiou, K., Guenet, B., Torn, M.S., Huang, Y., Zhang, H., Feng, W., Jagadamma, S., Kaiser, K., Kothawala, D. and Mayes, M.A., 2021. How much carbon can be added to the soil by sorption? Biogeochemistry, 152(2–3), pp.127–142.
Beerling, D. J., Kantzas, E. P., Lomas, M. R., Wade, P., Eufrasio, R. M., Renforth, P., Sarkar, B., Andrews, M. G., James, R. H., Pearce, C. R., Mercure, J., Pollitt, H., Holden, P. B., Edwards, N. R., Khanna, M., Koh, L., Quegan, S., Pidgeon, N. F., Janssens, I. A., . . . Banwart, S. A. (2020). Potential for large-scale CO2 removal via enhanced rock weathering with croplands. Nature, 583(7815), 242–248. https://doi.org/10.1038/s41586-020-2448-9
Boudinot, F.G., Dreyfus, G., Frederick, C. and Powers, Y., 2023. Enhanced Rock Weathering in the Global South: Exploring Potential for Enhanced Agricultural Productivity and Carbon Dioxide Drawdown.
Eufrasio, Rafael M., Euripides P. Kantzas, Neil R. Edwards, Philip B. Holden, Hector Pollitt, Jean-Francois Mercure, SC Lenny Koh, and David J. Beerling. “Environmental and health impacts of atmospheric CO2 removal by enhanced rock weathering depend on nations’ energy mix.” Communications Earth & Environment 3, no. 1 (2022): 106.
Haque, F., Santos, R. M., & Chiang, Y. W. (2020). Optimizing Inorganic Carbon Sequestration and Crop Yield With Wollastonite Soil Amendment in a Microplot Study. Frontiers in Plant Science, 11, 544852. https://doi.org/10.3389/fpls.2020.01012
Hartmann, J., Moosdorf, N., Lauerwald, R., Hinderer, M., & West, A. J. (2014). Global chemical weathering and associated P-release — The role of lithology, temperature and soil properties. Chemical Geology, 363, 145–163. https://doi.org/10.1016/j.chemgeo.2013.10.025
Hotchkiss, E. R., R. O. Hall Jr, R. A. Sponseller, D. Butman, J. Klaminder, H. Laudon, Martin Rosvall, and J. J. N. G. Karlsson. “Sources of and processes controlling CO 2 emissions change with the size of streams and rivers.” Nature Geoscience 8, no. 9 (2015): 696–699.
Jerden, James. “Crash Course on Enhanced Rock Weathering for Carbon Removal.” Remineralize the Earth, n.d. https://www.remineralize.org/2023/01/crash-course-on-enhanced-rock-weathering-for-carbon-removal/.
Kelland, Mike E., Peter W. Wade, Amy L. Lewis, Lyla L. Taylor, Binoy Sarkar, M. Grace Andrews, Mark R. Lomas et al. “Increased yield and CO2 sequestration potential with the C4 cereal Sorghum bicolor cultivated in basaltic rock dust‐amended agricultural soil.” Global Change Biology 26, no. 6 (2020): 3658–3676.
Lewis, A. L., Sarkar, B., Wade, P., Kemp, S. J., Hodson, M. E., Taylor, L. L., Yeong, K. L., Davies, K., Nelson, P. N., Bird, M. I., Kantola, I. B., Masters, M. D., DeLucia, E., Leake, J. R., Banwart, S. A., & Beerling, D. J. (2021). Effects of mineralogy, chemistry and physical properties of basalts on carbon capture potential and plant-nutrient element release via enhanced weathering. Applied Geochemistry, 132, 105023. https://doi.org/10.1016/j.apgeochem.2021.105023
McKinsey & Company. “A Blueprint for Scaling Voluntary Carbon Markets to Meet the Climate Challenge,” January 29, 2021. https://www.mckinsey.com/capabilities/sustainability/our-insights/a-blueprint-for-scaling-voluntary-carbon-markets-to-meet-the-climate-challenge.
Moharir, Dr. Kanak & Pande, Dr. Chaitanya & Patil, Sanjay. (2017). Inverse modeling of aquifer parameters in basaltic rock with the help of pumping test method using MODFLOW software. Geoscience Frontiers. 8. 10.1016/j.gsf.2016.11.017.
Rinder, T., & von Hagke, C. (2021). The influence of particle size on the potential of enhanced basalt weathering for carbon dioxide removal — Insights from a regional assessment. Journal of Cleaner Production, 315, 128178. https://doi.org/10.1016/j.jclepro.2021.128178
Strefler, J., Amann, T., Bauer, N., Kriegler, E. and Hartmann, J., 2018. Potential and costs of carbon dioxide removal by enhanced weathering of rocks. Environmental Research Letters, 13(3), p.034010.
Taylor, L. L., Quirk, J., Thorley, R. M., Kharecha, P. A., Hansen, J., Ridgwell, A., Lomas, M. R., Banwart, S. A., & Beerling, D. J. (2016). Enhanced weathering strategies for stabilizing climate and averting ocean acidification. Nature Climate Change, 6(4), 402–406. https://doi.org/10.1038/nclimate2882